Utilizing drone technology and orthomosaics as key tools for documentation and analysis.
by Hendrik Sass & Leonard Corves
(Tornado damage near Heere | ©TorKUD)
On June 18, 2024, severe thunderstorms developed across parts of Germany. In the southern Lower Saxony counties of Wolfenbüttel and Hildesheim, two supercells produced three confirmed tornadoes and one suspected tornado within just 35 minutes. Damage caused by these tornadoes was systematically documented using drones on June 20, 2024. Preliminary analyses were published by the end of June. This article examines the tornadoes, their impacts, and new methods in documentation and analysis, particularly emphasizing the advantages of drone technology and orthomosaic imaging for a comprehensive understanding of tornado dynamics.
(Overview of damage tracks in Hohenbüchen, Adenstedt (suspected tornado case), Bockenem & Heere)
Background
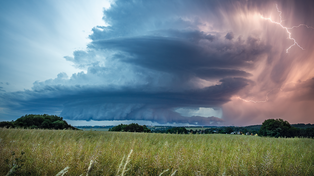
The dominant low-pressure system, named VALESCA, moved from the British Isles towards Scandinavia. Meanwhile, a secondary upper-level low-pressure system over northern Spain steered hot and humid air into southern and eastern Germany. Simultaneously, cool maritime air flowed into northwestern Germany, creating a distinct boundary between these air masses. This boundary was overlaid by a powerful jet streak from the southwest. Within the warm sector, several waves of severe thunderstorms and supercells developed from France to eastern Germany throughout the day. These storms produced significant impacts, such as large hail and severe downbursts, including a notable event in Gröditz, where large high-voltage towers were toppled. Additionally, during the afternoon, a small-scale disturbance along the boundary formed over North Rhine-Westphalia and advanced into southern Lower Saxony by early evening. This disturbance triggered new thunderstorms in southern Lower Saxony. Although the storms had limited energy due to thunderstorms that had traversed the region earlier in the day, kinematic conditions at the leading edge of the boundary were particularly favorable for tornado development. Additionally, preceding rainfalls contributed to increased atmospheric moisture, resulting in lower lifting condensation levels (LCL), which further supported tornado genesis. From these newly formed thunderstorms, several supercells emerged in southern Lower Saxony, which ultimately produced the tornadoes analyzed in this report.
(Soundings (reanalysis ensemble mean) in Hohenbüchen at 19:00 CEST & Heere at 20:00 CEST)
Radar analysis
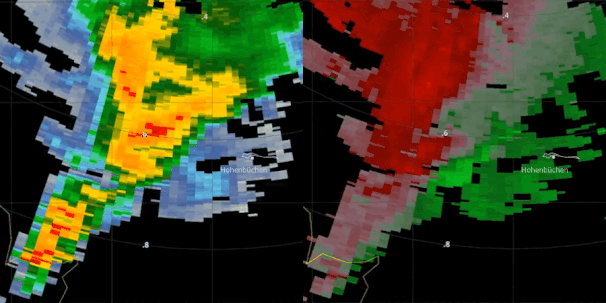
At 19:15 CEST, a small cluster of thunderstorms and rain showers entered the county of Hildesheim. The leading storm cell already displayed a compact rotation signature. By 19:35 CEST, this supercell produced the first tornado just north of Hohenbüchen, which traveled over a path of more than four kilometers. Shortly afterwards, a possible tornado was reported near Adenstedt around 19:45 CEST, followed by another confirmed tornado in Bockenem at 20:00 CEST. Notably, during the Bockenem tornado, no clear rotation was discernible on Doppler radar, even though the tornado maintained a continuous path of over six kilometers. Meanwhile, the northern storm cell increasingly developed the characteristics of a supercell. While the Bockenem tornado was still active, the rotation of the northern cell abruptly intensified, ultimately producing the strongest of the three tornadoes near Heere at approximately 20:10 CEST.
The radar analysis revealed that, contrary to initial assumptions, the event did not involve a single cyclic supercell but rather two distinct supercells. These findings align with the results of Nixon et al. (2024) regarding tornado genesis in adjacent supercells. Initially, the leading supercell produced at least two tornadoes, while the trailing cell remained underdeveloped. As the event progressed, the leading (southern) supercell began to weaken, not solely due to its own lifecycle but also because of the intensification of the trailing (northern) cell. This trailing cell gradually advanced into the foreground, likely exerting dominance over the atmospheric environment. It ultimately became the leading and northernmost supercell, producing the strongest tornado of the event shortly thereafter.
Documentation
After the first reports of the tornadoes appeared on social media on the evening of June 18, a field investigation was planned, which took place on June 20. The examination of the three confirmed tornadoes required about 12 hours. The journey to and from the site, covering nearly 1,000 kilometers, took a similar amount of time. In total, over 16 kilometers of continuous damage paths were surveyed, and 637 ground and aerial photographs were captured. Alongside the tornado in Hagen, which occurred three weeks earlier, this marked the first time that tornado impacts were systematically documented using a drone. This approach made it possible to efficiently examine an area of over four square kilometers from the air, enabling the documentation of three events of this scale within a single day. Moreover, the aerial imagery provided especially informative perspectives that would have been impossible to achieve with ground-based methods alone.
(Orthomosaic created from drone images of tornado damage on June 18, 2024 | ©TorKUD)
Damage images from social media were already being analyzed on June 18 and 19 to roughly map the affected areas. At that time, it was still unclear whether the event involved one or multiple tornadoes. Heere and Hohenbüchen were initially considered as suspected cases, as no video or photographic evidence of the tornadoes themselves was (and still is) available. Areas with buildings were prioritized during the investigation because such areas typically experience faster damage repair than rural areas, where damage remnants persist longer. Prominent damage sites were selected as starting points for the investigation because these locations appeared most likely to reveal continuous damage paths.
A drone equipped with multiple batteries and a power bank was utilized to ensure continuous flights, alongside a digital camera for ground-based documentation. The drone proved to be an essential tool for documenting field tracks and inaccessible damage sites, particularly in Heere and north of Hohenbüchen, where damage was located in forested areas or remote fields.
(Overview of on-site documentation & timeframes of the surveys | beige = tornado track; yellow = distance covered on foot, total > 20 kilometers; black = areas surveyed by drone)
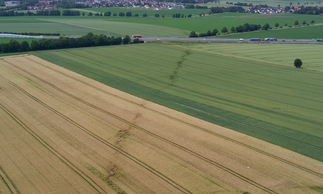
Without the use of drone technology, the results of the tornado investigation would have been significantly less accurate. For example, over 75% of the damage path caused by the Heere tornado was documented exclusively from the air because the path primarily consisted of field marks, which were invisible from the ground. These marks provided crucial insights into the tornado’s dynamics, including the formation of subvortices and wind directions. Similar examples were observed with the tornadoes in Hohenbüchen and Bockenem. For instance, without aerial documentation, the official path length for the Bockenem tornado would have been substantially underestimated. While no visible damage to property or vegetation was evident beyond Bockenem, a narrow field track extended well beyond the A4 Autobahn. Had this track remained undetected, the official path length would have been reduced by more than two kilometers.
Similarly, in Hohenbüchen, the most intense forest damage north of the town could only be documented using the drone, as the affected area was otherwise inaccessible. These examples underscore the critical role of drone technology in conducting thorough and precise on-site investigations. The use of drones has proven to be an indispensable tool for tornado documentation and will play a vital role in future investigations.
(Overview of on-site documentation | beige = tornado track; blue = sections of the track where impacts could only be effectively documented using a drone)
Key Insights from the Documentation Process
- The drone was crucial for documenting field tracks invisible from the ground and for accessing damage in difficult-to-reach locations, such as forests or remote fields.
- The integration of drone imagery with ground-level inspections proved essential for creating a complete and precise record of damage.
- Presenting credentials, such as identifaction cards, facilitated smoother operations and interactions with residents in the affected areas.
Analysis & Results
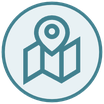
Following the completion of the on-site investigation, the recorded damage data were initially plotted on an interactive map using Google My Maps. This mapping process enabled the determination of central parameters, such as the total length of the damage tracks—measured from the first to the last observed impact—as well as the approximate maximum width. Additionally, this approach identified the areas with the most severe damage for each of the three documented tornado cases.
Determining Tornado Intensity
To provide a assessment of the tornadoes’ intensity, the most severe damage points were classified using the International Fujita Scale developed by the European Severe Storms Laboratory (ESSL).
Initial evaluations and accompanying photographs were shared with external experts, including Thomas Sävert (Tornadoliste.de) and Thilo Kühne (ESSL), for further review and discussion. This
collaborative evaluation process ensured a validated intensity classification on the IF scale for each tornado.
(Tornado damage north of hohenbüchen | ©TorKUD)
Hohenbüchen:
The most severe damage occurred just north of Hohenbüchen at the beginning of the damage track, within a mixed forest. As birch trees predominated in this forest, the damage was assessed using
the damage indicator TSW (Tree Stand, Weak), which applies to tree stands composed mainly of weak trees. Within the affected area, over 90% of the trees were uprooted or snapped, corresponding to
the highest degree of damage (DoD 4) for this indicator. Based on this damage, the tornado intensity at this point was classified as IF1.5
(TSW, DoD 4). It is worth noting that even the peripheral trees, which generally exhibit greater stability, were all toppled. Additionally, trees of sturdier species within the affected area were
entirely destroyed. While a slightly higher intensity (IF2) cannot be ruled out, this remains a borderline case. In such situations, the lower classification—IF1.5—should be adopted, as it is
conclusively supported by the evidence.
(Tornado damage at the K75 near heere ©TorKUD | center image: ©Feuerwehren der Samtgemeinde Baddeckenstedt | right image: before-and-after comparison ©TorKUD & ©Google Street View)
Heere:
The most significant damage occurred in the final third of the track along the K75, where substantial impacts were observed over a 400-meter stretch. A mixed forest west of the K75 suffered extensive damage, with large areas of trees either uprooted or snapped. Unlike in Hohenbüchen, this forest consisted primarily of tree species with average stability, making the TSA (Tree Stand, Average) damage indicator applicable. Aerial views from the orthomosaic clearly showed that over 90% of the trees in this section were destroyed, including peripheral trees. The remaining trees were mostly partially defoliated. This corresponds to the highest degree of damage (DoD 4), resulting in an intensity classification of IF2 (TSA, DoD 4).
When crossing the subsequent K75, three linden trees were significantly defoliated. A before-and-after comparison vividly illustrates how much crown volume was removed by the tornado. As linden trees are not classified as weak species, the damage indicator TRA (Tree, Average) was applied. Since well over one-third of the crown volume was removed without the trunk snapping—and only a few lower branches and leaves remained intact—the damage degree was assessed as DoD 6. This again corresponds to an intensity classification of IF2 (TRA, DoD 6), consistent with the previously identified IF2 damage.
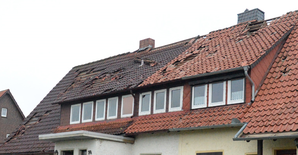
Bockenem:
The tornado caused extensive roof and tree damage in the towns of Bönnien and Bockenem. The intensity remained fairly consistent, ranging from IF0.5 to IF1. The pattern consisted of moderately damaged tiled roofs (strong tiled roofs, BNTS, DoD 1 = IF1), uprooted trees (both weak and average stability, TRW/TRA, DoD 3 = IF0.5 to IF1), and broken branches (mostly TRA, DoD 1 = IF0.5). The maximum intensity, therefore, was assessed as IF1.
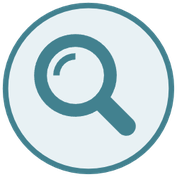
For each of the three tornadoes, a comprehensive analysis was published, detailing the development and intensity progression of the tornado tracks. Damage in various sections of the tracks was thoroughly described and evaluated according to the International Fujita Scale (IF scale). These analyses were complemented by accompanying graphics, including the precise path of the tornado tracks, wind directions, and key data such as track length and width, affected locations, potential injuries, and, where available, photographic or video evidence of the tornadoes. Analyses are intended for publication, although they include only a selection of damage photographs. All additional data and images are archived and made accessible to specific interested groups via a cloud platform.
Insights from the Use of Orthomosaics
A new element in the evaluations was the use of orthomosaics created from drone imagery, inspired by the Canadian Northern Tornadoes Project (NTP) at Western University. Drone imagery was
projected onto satellite images in Google Earth, enabling precise measurement and visualization of the tornado tracks.
(Orthomosaic of the Heere Tornado | white = area of impact; green = IF1; yellow = IF1.5; orange = IF2 | Drone images ©TorKUD & satellite images ©Google Earth)
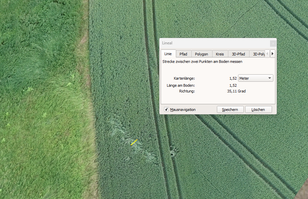
One central aspect is the ability to measure tornado paths with exceptional precision. A striking example of this is the Heere tornado, which left a field track less than two meters wide at the very beginning of its path. This track could only be accurately measured by overlaying the drone imagery onto satellite images in Google Earth. The alignment of the drone images with the satellite images ensured correct scaling, allowing precise measurements using the tools integrated into Google Earth. This facilitated accurate determination of parameters such as track width and length.
The tornado cases of Heere and Hohenbüchen were initially measured in June 2024 using maps based on images from the on-site documentation. The subsequent measurement using orthomosaics produced slightly differing but significantly more accurate values:
Heere
- Track length: 5,2 kilometers
- Maximum width: 375 meters (at the K75 road, where the most intense damage occurred)
- Minimum width: 1,5 meters (directly at the start of the track)
- Average width: 110 meters (averaged from 10 measurements, excluding extreme values)
Hohenbüchen
- Track length: 4,55 kilometers
- Maximum width: 370 meters (north of Hohenbüchen, directly at the start of the track)
- Minimum width: 30 meters (north of Gerzen)
- Average width: 130 meters (averaged from 10 measurements, excluding extreme values)
(Tornado tracks of Heere (left) and Hohenbüchen (right) | Initial measurement in June 2024 = yellow; Measurement using orthomosaics = red)
Tornado Dynamics and Wind Patterns
Both tornadoes underwent two distinct phases: one in which only a single primary vortex was discernible in the center of the track through field marks, and another in which multiple subvortices became visible, circling the central circulation (evident through cycloidal field marks). Both tornadoes transitioned from one phase to the other during their respective lifespans.
In Hohenbüchen, the track was already more than 200 meters wide at the beginning, and the presence of several pronounced subvortices was evident from the field marks, correlating with the highest observed intensity. As the tornado progressed, the track narrowed significantly, leaving only a classic primary vortex in the center of the track. At this stage, the intensity of the damage decreased considerably.
In contrast, the Heere tornado began with a narrow track, initially revealing only a single continuous vortex in the center. It was only in the second half of the path, east of the K75 road, that
multiple pronounced subvortices became visible, circling the main circulation. As in Hohenbüchen, the most intense damage and the widest part of the path occurred in the areas where cycloidal
field tracks formed.
The tornado that traveled through Bönnien and Bockenem, however, exhibited only a single primary vortex in the center of its path throughout its entire lifetime. Unlike the tornadoes in Hohenbüchen and Heere, no significant intensity peaks were observed. Such maxima in the analyzed tornadoes appeared to be associated with phases in which multiple pronounced subvortices replaced a single continuous vortex or when the transition to this phase was imminent.
(Orthomosaic of the Hohenbüchen Tornado | white = area of impact; green = IF1; yellow = IF1.5 | Drone images ©TorKUD & satellite images ©Google Earth)
(Wind field vVisualization - Heere Tornado | Blue arrows = treefall directions; black/gray arrows = field marks; red arrows = subvortices)
The sudden increase in intensity and width of the Heere tornado at the K75 road (see image above, right) may be attributed to a phenomenon known as a vortex breakdown. During this process, a bubble of extremely low air pressure forms several dozen meters above the ground, causing the collapse of the primary vortex, which then descends to the surface. This phenomenon typically results in the most intense damage, accompanied by an abrupt—albeit temporary—increase in the track width. The subsequent formation of multiple pronounced subvortices circling the main circulation, as observed east of the K75, is also characteristic of such a collapse. A visual example of this phenomenon can be seen in this YouTube video between 0:20 and 1:00.
(Left & center images: Wind field visualization - Hohenbüchen Tornado | Right image: Wind field visualization - Heere Tornado subvortices | Legend see previous image collage)
For the first time in German tornado history, such detailed field tracks were documented on this scale and precisely analyzed using orthomosaics. Particularly noteworthy is the clarity with which the subvortices could be identified from the distinct tracks. This was especially prominent in two areas: north of Hohenbüchen and east of the K75 road near Heere, where these patterns were precisely measured and visualized during the analysis (see images above). It is striking that the pronounced subvortices were primarily located on the left side of the track, while to the south of them, bulges with predominantly linear wind patterns were observed. In our cases, this suggests an intensified rear flank downdraft (RFD) when pronounced subvortices occurred. Furthermore, the behavior of the vortices could be clearly traced: they moved in a semicircular pattern around the main circulation before dissipating. No tracks in the form of closed cycloids were observed. The wind patterns indicate that radial winds predominated within these short-lived subvortices (Karstens et al., 2013).
(Pronounced subvortices at Heere (left image) and Hohenbüchen (center and right images) | ©TorKUD)
The work with orthomosaics proved not only to be successful in retrospect but also holds the potential to become a central component of future evaluation processes. Orthomosaics provide a standardized and scalable analysis format, enabling both precise measurements and visual documentation. However, as the documentation conducted on June 20, 2024, was not initially intended for the creation of orthomosaics, challenges arose during the subsequent assembly of the drone images. To facilitate the process in the future, the following framework conditions were established:
- The drone should be operated from greater altitudes and vertical perspectives.
- A larger number of drone images is essential to improve the quality and completeness of the orthomosaics.
- Documentation in a grid pattern would be advantageous but can only be performed manually, as our technology does not support this function. This would, however, involve significant time expenditure in our case.
Currently, no suitable platform is available to publish the orthomosaics as interactive maps. Nevertheless, the orthomosaics are accessible for download via a cloud to authorized interested parties.
Conclusion
The tornado events in southern Lower Saxony on June 18, 2024, represent a significant example of the application of advanced methods in tornado documentation and analysis in Germany. Through the targeted use of drones and the subsequent evaluation with orthomosaics, a detailed and precise recording of tornado damage was achieved, significantly complementing and expanding upon previous methods. In particular, the measurement of field tracks and the investigation of areas only visible from a bird’s-eye view provided deeper insights into the dynamics of the tornadoes.
Summary of Findings:
- The radar data analysis revealed that the event did not involve a single cyclic supercell but rather two adjacent cells that produced tornadoes and influenced one another, in accordance with findings by Nixon et al. (2024).
- The investigation of wind directions in the Heere tornado aligns closely with the formation and mature phases defined by Karstens et al. (2013).
- Both the Heere and Hohenbüchen tornadoes exhibited two distinct phases: one dominated by a central primary vortex and another featuring multiple subvortices circling the main circulation.
- Intensity peaks occurred when the tornadoes transitioned into or were already in the phase involving multiple pronounced subvortices. For the Bockenem tornado, which did not undergo this phase, no significant intensity increases were observed.
- The presence of multiple subvortices in the Heere and Hohenbüchen tornadoes was accompanied by an intensified rear flank downdraft (RFD).
- A vortex breakdown could explain the sudden increase in intensity and the widening of the Heere tornado’s track near the K75 road.
- The use of orthomosaics allowed for the re-measurement of tracks, resulting in updated, significantly more precise values for track parameters.
The evaluation process has clearly demonstrated that the use of drones in on-site tornado damage investigations is indispensable for precise and comprehensive analysis. Orthomosaics provide an accurate depiction of tornado tracks and enable precise measurements of damage areas, which were not feasible with previous methods. These approaches are therefore set to become a central component of future evaluation processes and are essential for the continuous improvement of tornado documentation.